Chaoborus and Hypoxia Effects on Reservoir Food Webs and Fisheries
Assessing drivers of prey availability to sport fish in Ohio reservoirs
Prey availability often plays an essential role in driving sport fish growth and subsequent recruitment.
The central goals of our project are to help improve the ability of the Ohio Department of Natural Resources-Division of Wildlife (ODNR-DOW) to:
- better understand the ecological mechanisms that might underlie seasonal variation in zooplankton availability to larval and juvenile (i.e., pre-recruited) sport fishes, and
- better assess prey-fish availability (i.e., gizzard shad, Dorosoma cepedianum) to larger top predators in Ohio reservoirs.
This project focuses on the role of a common macroinvertebrate, Chaoborus, in influencing the availability of both prey fish and zooplankton (ZP). Chaoborus migrate daily between dark hypoxic bottom waters in the day and more oxygenated surface waters at night where they feed on ZP.
This movement pattern is potentially problematic for two reasons:
First, Chaoborus hold great potential to reduce ZP prey availability to pre-recruited sport fishes (e.g., saugeye, Sander canadensis x S. vitreus; Pomoxis spp.; Lepomis spp.) by consuming ZP in the surface waters at night that would otherwise be available to sport fish. At present, a comprehensive assessment of whether Chaoborus limit ZP availability to pre-recruited sport fishes is lacking.
Second, because the gas bladders of Chaoborus resonate well at the hydroacoustic sampling frequency (~200 kHz) used by the ODNR-DOW when estimating prey-fish availability to larger predators at night, these estimates might be biased high by the presence of Chaoborus in the water column. At present, we have little understanding of the magnitude of this bias, including how it varies across reservoirs that differ in their physical (e.g., size, thickness of bottom hypoxic layer) and biological (e.g., Chaoborus and gizzard shad densities) characteristics.
We address both of these information gaps by accomplishing the following objectives:
- Determine how food web interactions and physicochemical conditions combine to influence prey availability to pre-recruited sport fishes.
- Quantify the bias introduced by Chaoborus into hydroacoustics estimates of available prey-fish biomass and develop a model to correct for this bias.
Objective 1: Determine how food web interactions and physiochemical conditions combine to influence prey availability to pre-recruited sport fishes.
We compiled and analyzed existing environmental, zooplankton (ZP), Chaoborus, and fish recruitment and growth data from previous collections and projects. We used this analysis to:
- Gain insight into patterns in ZP availability (abundance and community composition) during spring through summer
- Assess potential abiotic drivers of sport fish recruitment and growth
- Relate ZP availability to observed sport fish growth and recruitment success
We paired this retrospective analysis with data collected in a field study of three Ohio reservoirs (Alum Creek, Acton, and Burr Oak). The field study sought to test the potential for food web interactions to influence ZP availability to sport fishes in the absence and presence of hypolimnetic hypoxia. In this study we collected ZP, Chaoborus, fish, abiotic data, and hydroaccoustic survey data.
From the field data we determined ZP and Chaoborus abundance. We also used the hydroaccoustic data to determine Chaoborus and fish abundance. Finally, we quantified food web interactions in the laboratory by identifying and quantifying diets of Chaoborus, gizzard shad, and sport fishes.
Objective 2: Quantify the bias introduced by Chaoborus into hydroaccoustics estimates of available prey-fish biomass and develop a model to correct for this bias.
We collected data from six Ohio reservoirs (Hoover, Findlay #2, Acton, Alum Creek, Burr Oak, and Pleasant Hill) to inform the construction of a bias-correction model for the presence of different Chaoborus densities in prey-fish biomass estimates. We used discrete-depth pump sampling and whole-water column net tows to capture ZP and individual Chaoborus.
To build a robust bias-correction model, we sought to develop target strength (TS)-total length (TL) and TS-weight (W) relationships for gizzard shad during summer 2019. To build these predictive relationships, we conducted fieldwork over a 2-week period in Alum Creek Lake (similar to Brooking and Rudstam 2009). During the first day of each week, we used electrofishing to capture gizzard shad which were then kept in small net pens overnight. The following day, we set-up the hydroacoustics equipment and a large net cage (3 m long x 3 m wide x 8 m deep; 3.2-mm delta knotless nylon mesh). We added up to 20 gizzard shad (all of a single 10-mm size class) to the net cage and continuously insonified the individuals in the net cage to determine individual-shad TS with 70-, 120-, and 200-kHz transducers. Every 12 hours over a 24-hour period, we changed batteries connected to the hydroacoustics equipment and determined vertical profiles of temperature, dissolved oxygen concentration, and turbidity with a multi-parameter sonde (Model 6600, YSI, Yellow Springs, OH) and photosynthetically-active radiation with a light meter (LI-1800, LI-COR, Lincoln, Nebraska). We added 20 different shad into the net cage every 12–24 hours until four distinct 10-mm size classes of gizzard shad had been insonified (i.e., 40–49 mm, 50–59 mm, etc.). We repeated the insonification experiment the following week.
Objective 1: Determine how food web interactions and physiochemical conditions combine to influence prey availability to pre-recruited sport fishes.
Seasonal differences in food web structure
One goal of this research was to better understand the ecological mechanisms that might underlie seasonal variation in zooplankton availability to pre-recruited sport fishes. We focused on hypoxia as a primary driver of seasonal zooplankton availability and sought to answer the question: Does north-temperate reservoir food web structure differ from normoxia to hypoxia?
We hypothesized that hypoxia would result in greater overlap among the organisms in the food web (Figure 1).
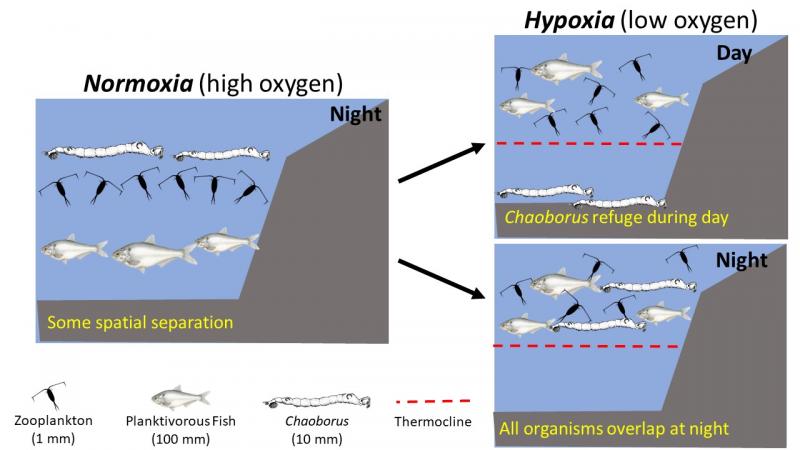
We found that while spatial overlap between planktivorous fishes, zooplankton, and Chaoborous is always high, it does tend to increase at night, especially during hypoxia (Figure 2). There is a high level of overlap at all times between planktivorous fishes and zooplankton, but that the level of overlap does indeed increase slightly during hypoxia at night. The level of overlap between planktivorous fishes and Chaoborus similarly increases at night during hypoxia.
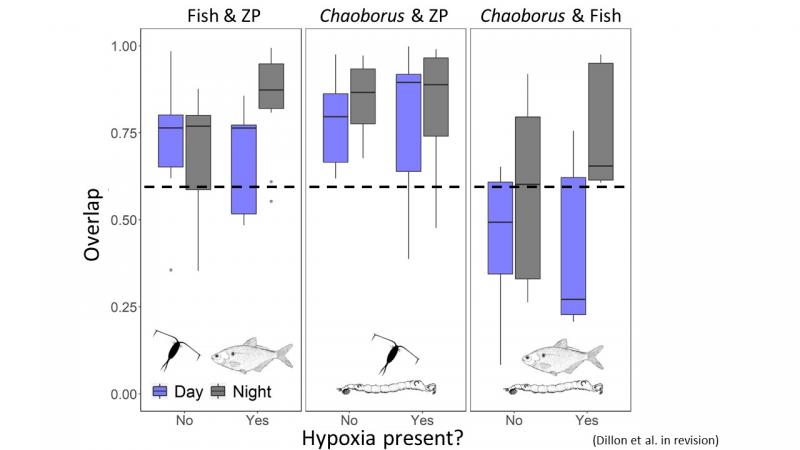
We also directly tested for differences in the abundance of organisms in oxygenated versus hypoxic portions of the water column. We found that planktivorous fishes and zooplankton were found significantly more in the well-oxygenated surface waters than in the hypoxic bottom waters. We also found that during the day, Chaoborus were found in the hypoxic bottom waters, likely as a refuge from predation, as they were the only organism found to reside in this portion of the water column. Chaoborus then moved into the well-oxygenated surface waters at night, where they overlapped with the zooplankton and planktivorous fishes.
This exploration of spatiotemporal overlap informs us generally that there is a potential for shifts in food web structure from normoxia to hypoxia. Next we seek to determine how this impacts larval sport fish.
Prey availability to larval sport fish
We sought to answer the question: How do hypoxia and intermediate consumers influence prey availability to larval sport fish?
To answer this question, first we explored the diet selectivity of Chaoborus and different sport fish larvae in the system and whether this selectivity demonstrated an overlap in diets between these organisms. We found that Chaoborus and the fish larvae all selected for copepods. We also found high levels of diet overlap. Overall, this indicates the potential for competition between Chaoborus and larval sport fish as consumers of zooplankton.
Next we explored whether zooplankton was ever limited in the system. We found that there is a potential for zooplankton limitation, and that this limitation is due Chaoborus consumption. We found that this potential limitation occurred only during summer, which is also the height of bottom hypoxia and the peak of Chaoborus population density.
The similar diet selectivity and diet overlap of Chaoborus and sport fish larvae as well as the potential for Chaoborus to limit zooplankton availability further enforces the idea that Chaoborus has the potential to impact sport fish access to prey, and impact the structure and function of the food web overall.
Objective 2: Quantify the bias introduced by Chaoborus into hydroaccoustics estimates of available prey-fish biomass and develop a model to correct for this bias.
The second goal of this research was to better assess prey-fish availability to larger top predators in Ohio reservoirs.
ODNR-DOW conducts annual hydroacoustic surveys in Ohio reservoirs to estimate prey fish abundance to get an understanding of food web structure. These surveys are conducted using 200 kHz transducers, and have been ongoing since the 2000s, but have not incorporated many recent advances in freshwater acoustic analysis.
Our data demonstrated that it is likely that there is bias in the ODNR-DOW hydroacoustic surveys because, using their methods, we found increasing densities of fish with increasing frequencies of hydroacoustic sampling (70, 120, and 200 kHz). If there was no bias, we would expect similar density estimates across all frequencies.
To determine what accounted for the increase in density estimates at high frequencies, we considered Chaoborus (which have high resonance at 200 kHz, Knudsen et al. 2006). We were able to estimate Chaoborus abundance in the high frequency hydroacoustic data by modifying an analysis protocol from Rudstam et al. (2008). We then matched our hydroacoustic estimate of Chaoborus to our estimate from discrete depth pump samples, and found a strong relationship between these two variables. This strong relationship indicates that the extra acoustic energy at high frequency transducers is indeed from Chaoborus, and that Chaoborus are responsible for the high bias in estimates of gizzard shad abundance using high frequency transducers.
In summation: We found that the presence of Chaoborus in the water column can bias estimates of fish density, especially when a high-frequency transducer (200 or 430 kHz) is used. The degree of bias is season-dependent due to changes in the demographics of Chaoborus. Bias can be limited by using a lower frequency transducer (70 kHz) and simple thresholding. It can also be limited by choosing an appropriate season for sampling - larger Chaoborus in the spring introduce more bias than the smaller Chaoborus found in the summer (Dillon et al. 2020).
- Dillon, R.A., J.D. Conroy, L.G. Rudstam, P.F. Craigmile, D.M. Mason, S.A. Ludsin. 2020. Towards more robust hydroacoustic estimates of fish abundance in the presence of pelagic macroinvertebrates. Fisheries Research, 230:105667. https://doi.org/10.1016/j.fishres.2020.105667
- Dillon, R.A., J.D. Conroy, S.A. Ludsin. 2019. Hydroacoustic data-analysis recommendations to quantify prey-fish abundance in shallow, target-rich ecosystems. North American Journal of Fisheries Management 39:270-288. https://doi.org/10.1002/nafm.1026